In the previous section (entrainment – 1) we saw how a single paced impulse can be used to entrain a re-entrant circuit.
The core pre-requisite of that maneuver is to capture the excitable gap – therefore it is critical that the pacing impulse be delivered in the excitable gap.
In real life, if the circuit is very large (e.g. AVRT) this is possible as we have other electrograms to predict the excitable gap. The classic example is using the His signal to see where the wavefront is. An impulse delivered at the RV apex will catch the excitable gap if it is delivered when the His spike is seen in the His catheter. (see section on His Synchronous PVC)
Unfortunately most other circuits do not have such facilities to predict the excitable gap and we need to figure out a way to get into the excitable gap.
That’s why most entrainment attempts are done with a train of paced impulses.
Also note that – EP Stimulators will deliver a single pacing impulse only after detecting the intrinsic beat + lapse of the pacing cycle length. For example if you set a paced stimulus at 300 ms, the system will deliver it 300 ms after detecting the intrinsic beat. The system does not randomly give paced beats – this is to prevent R on Ts and also to have some predictability of the procedure.
Lets look at a re-entrant circuit where the pacing electrode is right on the tachycardia circuit –

As shown in the above diagram, if you are continuously pacing, to entrain the circuit – you need to go slightly faster than the tachycardia cycle length. This enables repetitive capture of the excitable gap. But why do we need to do this ?
The main reason is, that in most real world cases, we are not within the circuit and we need to penetrate in to the excitable gap by traveling through other tissue.
To understand this, lets look at a circuit whose native cycle length is 400 ms and the pacing electrode away from the circuit –
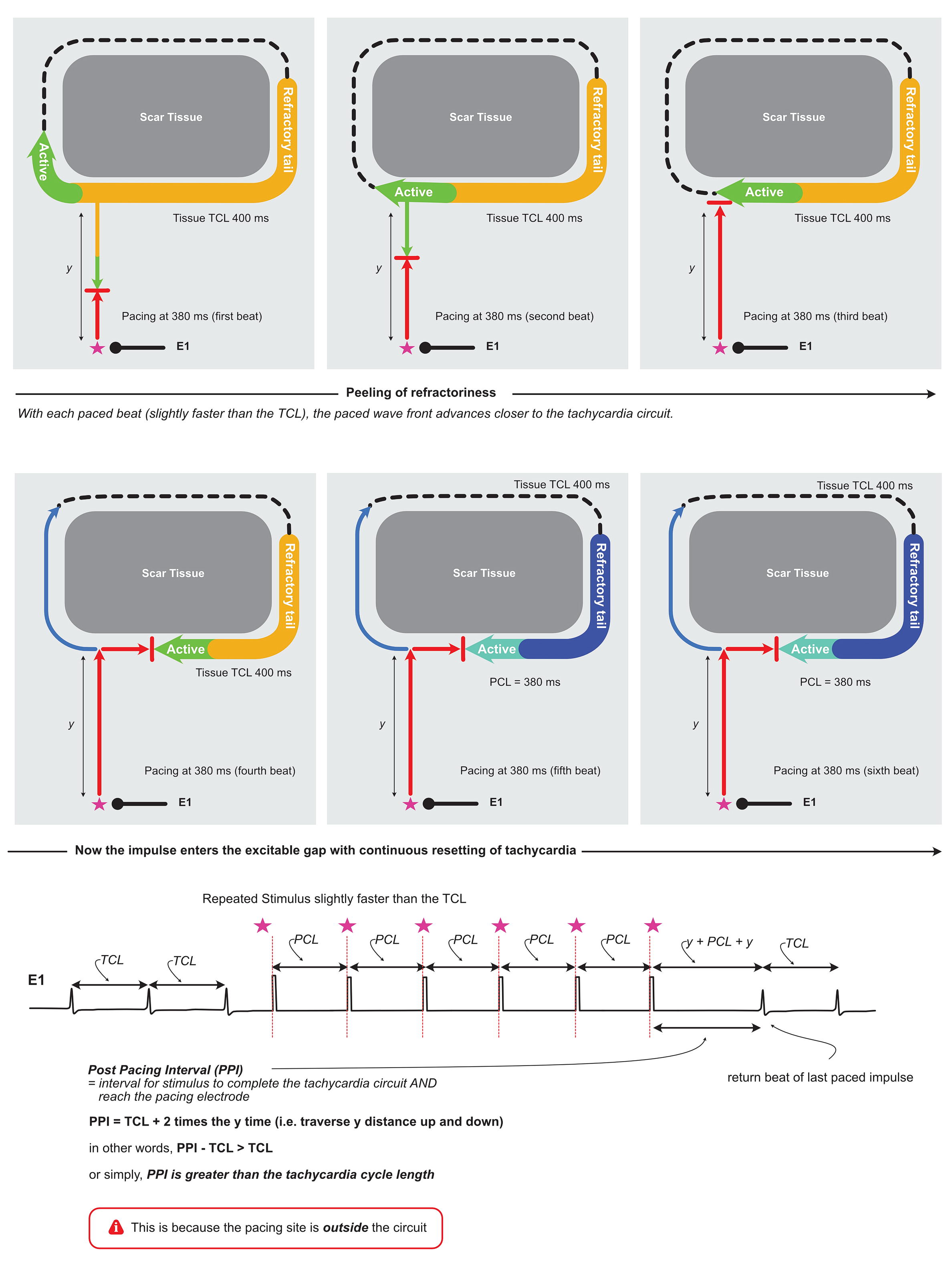
As explained in the above diagram, continious pacing at a rate slighhtly faster than the TCL enables us to penetrate in to the excitable gap. This is the fundamental method of entrainment of most re-entrant tachycardias.
Now, another big question – how do we know at what point in our pacing train that the tachycardia has been entrained ? For this we need to demonstrate that the wavefront traveling in the circuit is as fast as the pacing rate – in other words, the tachycardia “was accelerated” to the pacing rate.
On review of the above diagrams, it is apparent that the actual tachycardia speed is not changed (as the circuit is the same) its – just that we enter to it in the excitable gap and cause collision with the antidromic wavefront and propagation as the orthodromic wavefront. But if we have an electrode on the circuit, once the pacing has penetrated the circuit, the electrogram recorded on the electrode would occur at the pacing rate. In other words, any electrode in the circuit would register electrograms at the rate of pacing
Another marker that we can use is the surface ECG – this is called fusion – this also suggests entrainment – this is discussed in detail in the next section (Entrainment – 3)
The commonest practical way is to use another electrode – which is presumably in the circuit to confirm entrainment.
A good example, is entertainment of atrial flutter. Acceleration of the electrograms in catheter (e.g. halo catheter) which is lying in the circuit to the paced rate confirms entrainment of the flutter circuit. In a similar manner, ventricular pacing entrains an AVRT with acceleration of the atrial signal to the paced rate.
Another pointer – this is of theoretical interest. If the y distance in the above diagram is more than the tachycardia cycle length, we would not be be able to entrain the tachycardia consistently as the paced beats may not reach the excitable gap on time.
This looks like a problem when pacing from a far away site – e.g. pacing from the RV apex to entrain AVNRT. In this situation, The pacing waves have to reach the atria via the His – Purkinje system.
Fortunately, conduction in the His-Purkinje system is very fast (50 ms) – which gives a total travel time under 100 ms to reach the circuit of AVNRT. The typical cycle lengths of AVNRT is way more than 100 ms – and that’s why we can entrain AVNRT from the RV apex – despite the anatomic distance – the His purkinje system acting like a fast conductor
In the next section (Entrainment – 3) we will visit the concept of fusion and go thru the concepts behind “criteria for entrainment”